Quantum computing, a revolutionary field that began as a theoretical concept in the early 1980s with physicist Richard Feynman, is rapidly moving from labs into reality. Today, companies and researchers worldwide are accelerating efforts to harness its extraordinary power.
But what exactly is quantum computing, explained in simple terms? How does it differ from the computers we use daily, and what makes it so incredibly powerful for certain tasks?
In this beginner’s guide to quantum computing, we break down the basics of quantum computing into 10 essential facts. You’ll gain a clear understanding of how quantum computing works, its core principles like qubits explained, and the fundamental differences between quantum vs classical computing explained. Whether you’re new to tech or simply curious about this cutting-edge technology, this listicle offers a simple introduction to quantum computing and why it’s such a big deal.
10. It’s Not Just Faster—It’s a Whole New Kind of Computing
Quantum computers aren’t just supercharged versions of your laptop—they’re fundamentally different. They operate using the rules of quantum mechanics, the strange physics that governs atoms and subatomic particles. Instead of using bits (which are either 0 or 1), quantum computers use qubits, which can be both 0 and 1 at the same time. This unique approach makes them powerful for solving complex problems that regular computers simply can’t handle.
9. Superposition is Key
Superposition allows a qubit to be in a combination of both 0 and 1 simultaneously. Imagine you’re trying to pick a movie to watch. Instead of choosing just one, you get to watch all the movies at once—and not just that, you’re also able to understand and enjoy each one fully at the same time! You don’t have to pick between genres; you experience all the possibilities together. This is how superposition works in quantum computing: it lets quantum computers explore and process multiple solutions at once, allowing them to solve problems much faster than classical computers.
8. Qubits Are the Building Blocks of Quantum Computing
A qubit (short for quantum bit) is the most basic unit of information in a quantum computer. Unlike a classical bit, which is either a 0 or a 1, a qubit can be 0, 1, or both at once—thanks to the quantum principle of superposition. This allows quantum computers to perform multiple calculations at the same time, giving them a major edge in solving complex problems.
7. Entanglement Links Qubits—Even Across Space
Quantum entanglement is one of the most mind-bending concepts in quantum computing. When two or more qubits become entangled, they behave as a single, connected system—even if they are physically far apart, like on opposite sides of a room, or even the planet. This means that changing or measuring one qubit instantly affects the others, regardless of the distance between them. That’s why Einstein famously referred to it as “spooky action at a distance.” This phenomenon is critical for many quantum computing applications, including quantum communication and error correction.
6. Power Comes from Parallelism—Quantum Style
The real strength of quantum computing lies in its massive parallelism. Thanks to superposition and entanglement, a quantum computer can represent and work through many possibilities at once. With just a few hundred entangled qubits, a quantum machine could handle more quantum states than there are atoms in the known universe.
Example of Quantum Computing Parallelism
Imagine trying to crack a password made up of 10 letters and numbers—that’s over 60 trillion possible combinations. A regular computer, depending on its processing power, could take days, weeks, or even years to test every single combination one by one.
A quantum computer, using superposition and quantum algorithms like Grover’s algorithm, could reduce the number of steps needed from 60 trillion to roughly 8 million—a quadratic speedup. That means, in theory, a quantum computer could solve the same problem in minutes or hours, rather than years.
This ability to explore many possibilities simultaneously is what gives quantum computing its enormous potential in fields like cryptography, search, and optimization.
5. Quantum Computing vs Classical Computing
Classical computers use bits (0 or 1) and handle tasks step by step. Quantum computers use qubits, which can be in multiple states at once—allowing them to explore many possibilities simultaneously.
How They Process Information In short:
Classical = One step at a time.
Quantum = Many possibilities at once.
Despite all the hype, quantum computers aren’t built to replace your phone or laptop. You won’t be checking email or browsing the web on one. Classical computers are still faster, cheaper, and far more practical for everyday use.
Instead, quantum computers are designed for extremely complex problems—like simulating molecules for drug discovery, optimizing supply chains, or breaking encryption—that even supercomputers struggle to solve.
4. Potential Applications Are Transformative
Quantum computers have the potential to revolutionize multiple industries by solving problems that are currently too complex for even the most advanced classical computers. Here are some of the areas where quantum computing could have a transformative impact:
Artificial Intelligence: Quantum computing could significantly accelerate machine learning algorithms, potentially making AI more efficient and powerful.
Drug Discovery & Materials Science: Quantum computers can simulate molecules and materials at the quantum level, enabling the design of new medicines and materials with greater precision.
Optimization: Quantum computing excels at solving complex logistical problems, such as optimizing delivery routes, improving supply chains, and financial modeling—tasks that take traditional computers too long to solve.
Cryptography: Quantum computing can break current encryption methods (like RSA) but also offers the potential for quantum encryption, creating ultra-secure methods of protecting data.
This is all made possible by quantum computing’s ability to calculate multiple possibilities simultaneously, a capability that vastly outpaces classical computers when it comes to solving complex, real-world problems
3. Error Correction is a Major Hurdle
One of the biggest challenges in quantum computing is that qubits are extremely fragile and easily prone to errors. Due to their sensitivity, quantum error correction requires a significant number of extra resources. To create a stable, usable ‘logical’ qubit, many physical qubits might be needed. This extra overhead to maintain qubit reliability is one of the biggest engineering challenges currently faced by quantum researchers. Until effective error correction methods are developed, scaling quantum computers to practical levels will remain a major obstacle.
2. They Are Extremely Expensive and Difficult to Build and Operate
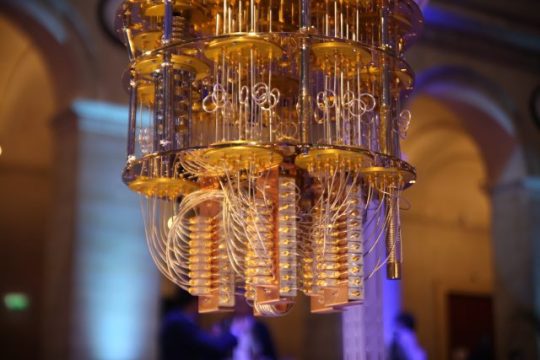
Qubits are highly fragile and easily disturbed by their environment. Even small changes in temperature, vibrations, or stray radiation can cause them to lose their quantum state—a phenomenon known as decoherence. This makes building and operating quantum computers a huge challenge. To maintain the delicate quantum state, quantum computers often need to be kept in highly controlled environments, such as at near absolute zero temperatures and shielded from any interference. These requirements make quantum computing not only complex but also costly to develop and maintain.
The quantum computer price can vary significantly, with the cost of building a fully functioning quantum system estimated at anywhere from US$15 million to US$50 million or more—depending on the system’s scale and the level of sophistication required.
1. It’s Still Early Days for Quantum Computing
Despite the incredible progress and excitement surrounding quantum computing, we are still in the early stages. Large-scale, fault-tolerant quantum computers—those that can be widely used for practical applications—are likely still years or even decades away. Currently, quantum computing is in what’s known as the era of Noisy, Intermediate-Scale Quantum (NISQ) devices. These devices are valuable for research and experimentation, but they’re still limited in both capability and reliability.
As the technology matures, however, the possibilities will expand significantly. In the future, when quantum computers become more advanced, they could have a profound impact on fields like healthcare. Quantum simulations could help researchers model the interactions between molecules, proteins, and drugs at a level of detail never before possible. This might pave the way for breakthroughs in understanding diseases like cancer and AIDS. Quantum computing could help us design personalized treatments, accelerate drug discovery, and even optimize the development of vaccines and gene therapies, potentially leading to faster cures for these complex diseases.